A reader writes:
Why do nuclear power stations (and other power stations, for that matter) have cooling towers in that weird half-hourglass shape?
I presume the guys who built them knew what they were doing, but what did they know that I don't?
Ian
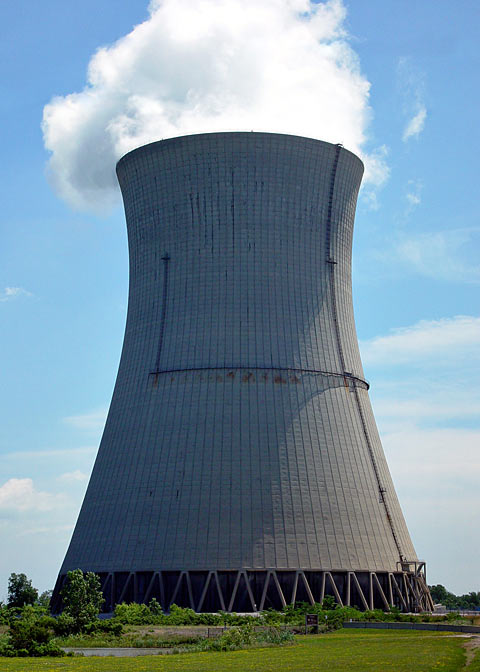
I pledge to eventually answer your question, Ian, but first I'm going to rabbit on interminably about power stations.
The cooling tower has become emblematic of nuclear power stations, and the white "smoke" drifting from the top of them is a source of vague nervousness for a lot of people.
But, as you say, other kinds of power stations have cooling towers too. I live less than an hour's drive from Lithgow and the Mount Piper and Wallerawang Power Stations, able to produce 3.4 gigawatts of coal-fired electricity between them; Mount Piper has two cooling towers, Wallerawang has one. The "smoke" that comes out of these towers is actually just clouds of tiny water droplets.
(Once again, if you can see it, it's not "water vapour". Clouds, and the visible "steam" squirting out of a kettle or a steam locomotive, are liquid water droplets with a ceiling temperature of 100°C at sea-level air pressure. It's possible for actual invisible-vapour steam to be swirled in with condensed droplets as it mixes more or less chaotically with the outside air, but "pure" steam is invisible, and has no ceiling temperature. Put your hand in the visible portion of the steam coming out of the side of a locomotive and you may get scalded, but putting your hand in the invisible jet close to where it's exiting may flense the flesh from your bones.)
Power stations need cooling towers, or some other heat-sink like water from a convenient river, because they are heat engines. Heat engines, as I've written before, become more and more effective as the temperature difference between their "hot end" and their "cold end" increases.
A heat-engine that makes this fact obvious is the now-quite-standardised sort of "coffee cup" Stirling engine...
...which stands on a wide circular displacer-piston cylinder and can run on the heat from a cup of coffee or tea, or backwards on a cup of ice-water. I've got one that runs like this, but really low-friction versions of the design can run on the heat from a human hand, if the ambient temperature is cool.
(You can pay quite a lot of money for a jewel-like Stirling engine {or, more interestingly, a kit to build one}, but this eBay dealer, in addition to being called "Stirlingeezer" which ought to be a reason to buy from him all by itself, sells quite beautiful engines and kits that are guaranteed to run from hand-heat. If enough people buy stuff via the above affiliate link to Stirlingeezer, I shall soon be able to afford one of his engines!)
(Oh, and if you're short of money, you can get a Stirling kit for $US30 delivered, or conceivably less if you get lucky with your bids, from this guy in China.)
Conventional power stations, whether fired by coal, combustible gas of one kind or another, or a nuclear reactor, make their electricity by turning a turbine connected to a generator. Gas-fired stations can do this directly with a gas turbine, which is essentially a jet engine tuned for shaft-turning power, rather than thrust. Coal and nuclear stations make electricity less directly, by using the heat of combustion or nuclear fission to boil water and run a steam turbine.
(I think there are also gas power stations that use steam turbines. There are definitely gas power stations that burn the gas in one turbine, and then run another, different turbine from the hot exhaust of the first one.)
Anyway, that's the hot end. A well-designed heat engine will try to get its cold end as distant in temperature from the hot end as is practically possible. The ratio between the two temperatures, expressed in Kelvin (or any other temperature scale, as long as it starts at absolute zero), determines the maximum possible efficiency of a heat engine.
Sometimes "the cold end" is synonymous with "the exhaust temperature"; that's how it works for internal-combustion piston vehicle engines, and steam engines too. A classic example of the latter is the triple-expansion compound steam engine. This has one small piston for the fresh, hot, high-pressure steam right out of the boiler. The medium-heat, medium-pressure exhaust from this first piston powers a medium-sized piston, and the low-heat, low-pressure exhaust from that piston in turn runs one or more even bigger pistons. (This can theoretically be extended to even more stages, but in practice quadruple-expansion was about as far as anyone could get before the gain in efficiency wasn't worth the extra complexity and friction.)
Steam-turbine power stations, on the other hand, may emit exhaust gases from the burning of fossil fuels, but the system that makes the actual electricity is a closed, Rankine-cycle steam/water circuit. The burning fuel or fissioning atoms heat cool water to steam, the steam turns a turbine or three, and the turbine exhaust then goes to some sort of cooling device, generally a heat exchanger, that dumps the final unusable portion of the water's heat somewhere.
This "somewhere" can be a separate water supply, either a river, large lake or sea, or it can be evaporating water in a cooling tower. Once the heat exchanger has cooled the closed system's water in whichever way, that water is pumped into the boiler again, and the cycle continues.
You might wonder why you need to dump heat from the turbine exhaust, when you're only going to heat the water up again in the boiler. There are two practical reasons for this.
The first reason is that the exhaust from a power turbine is almost all still water vapour, because, in brief, turbines made to run on a flow of hot gas do not like it if the gas condenses to liquid inside them.
The second reason is that the pump that returns the water to the boiler has the opposite preference; it only works with liquid water. It would be possible to use a gas pump instead and make a system in which the working fluid is always vapour, but the energy needed to run a gas pump against pressure from the boiler is high, while the energy needed to run a water pump is trivial (by power-station standards), on account of the incompressibility of the water.
The upshot of all this is that standard 20th-century power stations are pretty miserably inefficient. Today, there's much more effort being made to reduce the heat wasted, by for instance transferring some of the heat of the turbine exhaust to the water feed between the pump and the boiler, or by using some of the waste heat to keep nearby buildings warm ("cogeneration"). These sorts of measures can only go so far, though, so cooling towers of one shape or another will continue to be built.
Which, finally, brings us back to the classic cooling-tower shape.
Cooling towers actually come in all shapes and sizes; large air conditioners, for instance, often have evaporative coolers for their chillers, but those coolers don't look anything like a power-station cooling tower.
Power-station coolers have to have very large capacity, so they inescapably have to be very large. Power-station coolers also have to provide a decent convective "stack effect", also known as "draught" (or "draft", in the less-demented American spelling). But, importantly, power-station coolers don't really need to be able to hold up much more than their own weight, plus any remotely plausible wind loads or shifts of their foundations.
The classic curvy cooling-tower shape fits all of these requirements. In engineering terms, because cooling towers don't need to hold up an interior full of offices, they can be built as a "thin-shell structure". You could build a cooling tower out of giant Great-Pyramid stone blocks if you wanted to, but a surprisingly thin reinforced-concrete shell, built in layers from bottom to top (not unlike the way 3D printers work), is the usual solution. And the builders almost never balls it up.
Objects of this shape are called "hyperboloid structures"; they're strong for their weight and so have been used for all sorts of masts and towers and, sometimes, ordinary buildings too, and they're particularly suited for use as cooling towers. The large area at the bottom of the hyperboloid gives lots of room for evaporation, the "waist" accelerates the gas mixture (I think because of the venturi effect), and then the widening opening at the top encourages turbulent mixing with the ambient air. (Air gets into the tower in the first place via an open latticework section around the base.)
(Oh, and I just have to take a moment, here: Segmentally Constructed Prestressed Concrete Hyperboloid Cooling Tower! Segmentally Constructed Prestressed Concrete Hyperboloid Cooling Tower! Segmentally Constructed Prestressed Concrete Hyperboloid Cooling Tower! Thank you.)
The final question that occurs to me in this area is why cooling towers are hyperboloids, but factory chimneys are cylindrical (or close to it - they often taper a bit toward the top).
This is because the cooling tower wants to move a vast amount of low-pressure air. The evaporating warm water at the bottom of the tower produces a steam/air/water mixture that isn't much warmer, and thus less dense, than the ambient air, so it has little buoyancy compared with the ambient air, won't move terribly fast, and so has to pass through a really wide pipe. Factory chimneys, on the other hand, are moving a much smaller volume of much warmer gas, usually combustion-product "flue gas". This is usually quite a lot hotter than ambient, so it rather wants to go up a chimney and doesn't need a wide one; you just need a nice long chimney, both to get a strong stack effect and to discharge the gas as high up as possible, to spread the pollution by dilution, as it were.
(Incidentally, The Secret Life of Machines addresses the stack effect in episode five, on central heating. And while I'm on the subject, the extraordinary documentary Fred Dibnah, Steeplejack features the titular working-class hero climbing hundreds of feet up a brick chimney and then perching on scaffolding that looks as if it were assembled by blind drunkards and knocking the chimney down by bashing bricks, one by one, into the flue. It has to be seen to be believed.)
Psycho Science is a regular feature here. Ask me your science questions, and I'll answer them. Probably.
And then commenters will, I hope, correct at least the most obvious flaws in my answer.